Table of Contents
We have known for centuries that we share our bodies with microbes and that our resident or “commensal” microbes may be beneficial to our health. There are thousands of different species of microbes, including bacteria, viruses, and fungi/yeast, that inhabit our bodies (Martinez-Guryn 2019, Musumeci 2022). Most of them live in our guts (mouth to anus) and by far the largest population lives in the colon. But we also have microbes on our skin, in our lungs, and other internal structures. We may have a few microbes in our brains.
Only in recent years have we discovered just how critical having a healthy population of microbes is to our mental and physical health. Problems with microbes are hallmark features of gut disorders, including irritable bowel disorders, inflammatory bowel disorders, celiac disease, and food allergies. Beyond the gut, microbes can influence things like mood, cognition, and even brain development (Bastiaanssen 2019, Dickerson 2017, Dinan 2015, Gonzalez-Aranciba 2019, Kelly 2015, Koopman 2017, Nguyen 2018, Rees 2018, Yang 2019). Further, many different laboratories have now reported effects of microbes in the gut that go beyond brain effects (de Morales 2017, McCoy 2018), demonstrating that when gut microbial populations are disordered there are health consequences (Weiss 2017). It is becoming increasingly clear that the condition of our microbe communities markedly influences not just the health of our guts, but also our brains, metabolic, cardiovascular, and immune systems.
What is life in the gut like for microbes?
The gut is a hollow tube and microbes mostly live along the layer of cells, called the epithelium, that separates the inside of the tube (lumen), and the inside of our bodies. The epithelium is covered by mucous, which helps protect it. Some microbe species help maintain the integrity of the mucous layer, and this is an important way that microbes support gut barrier function. The gut barrier serves to absorb nutrients that have been digested and to keep out toxins and pathogens. That this is extremely important seems obvious, but recent findings have implicated impaired gut barrier function (which is sometimes called “leaky gut”) in causing or contributing to a wide variety of disease conditions, including liver diseases, gut disorders such as inflammatory bowel disease, allergies, and neurological conditions (Camilleri 2019).
The many different kinds of microbes communicate with each other, and they can influence each other’s growth. This seems to help protect against any one species overgrowing, which can cause symptoms such as gas and bloating or pain, and lead to disturbed gut barrier function. An example of overgrown microbes is small intestinal bacterial overgrowth (SIBO). Resident gut microbes (also called “commensal” microbes) may also help protect us from pathogenic microbes, such as those that cause food poisoning (Rogers 2021).
Our gut microbes are functionally part of “us”
We and our microbes exist in a symbiotic relationship, providing mutual benefit. We provide food and housing for them, and they, for example, provide us with beneficial nutrients, such as vitamin K and short-chain fatty acids like butyrate, a nutrient source for the gut epithelial cells.
This relationship is an active one, in that we communicate with them using the same hormone and neurotransmitter signals that our cells and tissues use to communicate within our bodies. For instance, bacteria respond to challenge/stress hormones such as norepinephrine, cortisol, corticotropin-releasing hormone (CRH) (Lyte 1992, Lyte 2014, Sarkodie 2019), and gonadal steroid hormones including estrogens (Vom Steeg 2017). These substances influence bacterial behavior and growth (Lyte 1992, Moriera 2016). For instance, both norepinephrine and cortisol, which are part of the challenge/stress response, increase the growth of microbial colonies and increase the virulence of the microbes, making them behave in aggressive ways that may be damaging to our health. This hormonal influence on our microbes could be one way that stress increases susceptibility to diseases such as gastrointestinal infections or inflammation and dental diseases.
Our interactions with our microbes are not “one-way streets”. Gut bacteria produce many of the same signaling and metabolic substances that we do, including neurotransmitters such as serotonin, GABA, acetylcholine, and dopamine (Margolis 2021, Roschina 2016), as well as short-chain fatty acids and amino acid metabolites. These substances are known to interact with cells of our bodies (Margolis 2021), including neurons. For this reason, gut microbes can be thought of as a “microbial endocrine organ” (Lyte 2016). In this way, microbes have a close and bidirectional relationship with our own cells, including brain cells.
Substances such as neurotransmitters that gut microbes produce can interact with “interoceptive” gut-brain pathways. Interoceptive nerves, including sensory nerves of the vagus nerve, provide information about the condition of the gut, such as the presence of food, or inflammation. Brain networks that are influenced by interoception influence motivation, help regulate emotions and even construct our sense of self. This means that microbes can, indirectly, influence our psychological functioning. To that end, some probiotic foods and formulations have been reported to improve mood and stress, further emphasizing the connections between microbes, gut, and brain (Bastianssen 2019, Koopman 2017, Langgartner 2019).
Microbes may provide missing pieces of the puzzle of psychiatric and neurological diseases
Although the symptoms and correlates of brain-related diseases, such as Alzheimer’s Disease, Schizophrenia, Autism, and mood disorders, have been recognized for many years, we are still frustratingly far away from understanding just what causes them and how to reliably treat or cure them. Many different factors, such as genetics, life history, and acute stress somehow interact to contribute to the causes and persistence of these conditions, resulting in rather individual experiences and etiology. It has seemed though, that some pieces of the puzzle of brain disorders have been missing, and decades of research have occurred without solving gaps in treatment for many psychiatric disorders. Recent studies now link disturbances in gut microbes to brain-related disorders (Dickerson 2017, Firth 2019, Fitzgerald 2019, Margolis 2021, Nguyen 2018).
One key clue about the pathophysiology of all of these disorders is either systemic inflammation or neuroinflammation (Firth 2019, Maes 1995), raising the question of what is driving this persistent inflammation. Several lines of evidence now support a role for gut microbes.
How could gut microbes drive inflammation?
One key mechanism may follow from gut barrier dysfunction. A “leaky” barrier can drive inflammation, and gut microbes play key roles in keeping it healthy, as we will see.
Further, the condition called dysbiosis, which describes the situation in which microbial populations are unbalanced (too many of some and not enough of others) and some can become aggressive, contributes to gut barrier impairment and low-grade inflammation. Indeed, gut dysbiosis is consistently associated with brain disorders (Firth 2019, Kelly 2015, Koopman 2017, Li 2017, Rea 2016), including Alzheimer’s and Parkinson’s Disease, schizophrenia, autism, and mood disorders.
Some studies have reported that probiotics can reduce depressive symptoms, promote resilience, and mitigate elevated cortisol during chronic stress (Park 2018). Taken together these observations support the idea that gut microbes are important factors in brain-related conditions Indeed, perhaps the most surprising thing about our relationships with our microbes involves the influence they seem to exert on our minds.
Imbalanced microbes: loss of “microbial diversity” leads to dysbiosis
One of the main goals for research about the patterns of microbes in the gut is to determine whether certain microbes or populations can improve gut health or do they lead to disease conditions. So far, the main finding is that having a larger number of different species (microbial diversity) seems to be the most important single indicator of a healthy microbial population. Some of the microbe species in our guts can act as pathobionts, which are resident microbes that can contribute to disease, but only if they overgrow, as well as other species that are clearly beneficial. Having lots of different species helps keep them from overgrowing.
When microbial diversity is low, it predisposes to dysbiosis, with the overall population of microbes being imbalanced. Symptoms of dysbiosis can include bloating, because of the excess gases produced by the overgrown bacteria, and pain.
Perhaps the most serious consequence of dysbiosis is disruption of the gut barrier, leading to increased permeability or “leaky gut”. This can cause a wide range of symptoms. Notably, these symptoms can include neurological experiences such as mood disorders, fatigue and cognitive “fuzziness”, as well as food, skin and respiratory allergies. Together these may contribute to poor quality of life and increased risk of serious illnesses, such as diabetes, inflammatory bowel diseases, cardiovascular disease, and even neurological disorders (Rinninella 2019, Weiss 2017).
One well-characterized example of severe dysbiosis is infection/overgrowth with Clostridium difficile (C. difficile). The condition is usually treated with powerful antibiotics, because C. difficile tends to be resistant to many other antibiotics. Unfortunately, people who have antibiotic-resistant C. difficile overgrowth can be treated with so many powerful antibiotics that nearly the only remaining species is C. difficile. This situation is life-threatening, because it can lead to severe colitis. Up until a few years ago, there was no reliably effective treatment. But there is now a revolutionary treatment that is saving the lives of people hospitalized with C. difficile.
What is this treatment? It is called “fecal transplant” and involves giving people with C. difficile overgrowth feces from a healthy person, either via enema, endoscopy, or pills. This typically leads to a cure within a few days, underlining the critical role of balanced microbial communities in maintaining gastrointestinal health.
Beyond bacteria- the mycobiome
Although most research on microbes and health has focused on the types of bacteria in our guts, recent work indicates that species of fungi also contribute benefits to gut health. This “mycobiome” seems to exist in a balance with bacteria (Jiang 2017, Patterson 2017). For instance, overgrowth of fungi, causing the condition called “thrush”, is common with antibiotic use. Probiotic yogurts can help (Kumamoto 2021). Conversely, fungi can compensate for some of the side effects of antibiotic use that are secondary to the loss of specific bacteria (Jiang 2017). Thus, the different kinds of microbes, when in balance and not overgrown, may be working in concert to help maintain gut health. This is a developing area of research that promises to provide important practical information relevant to keeping a healthy balance of microbes.
The correlation of low microbial diversity and dysbiosis with a wide variety of disease conditions has inspired research into the questions of what exactly microbes are doing to support health, and what are the mechanisms by which having too few can enable disease. Already it is clear that microbes play critical roles in regulating inflammation and tolerance to foods, responses to stress, and maintaining gut barrier integrity. How do they do that?
“Old Friends” regulate inflammation and help maintain tolerance to foods.
This association of low microbial diversity with poor health outcomes suggests that the “missing microbes” may be playing critical roles in our health. The association of the “cleanliness” of the urbanized Western lifestyle with allergy and autoimmune disease has given rise to the “Hygiene Hypothesis”, which has been refined into the “Old Friends Hypothesis” (Rook 2014). The idea is that urbanized lifestyles lead to reduced diversity, and in particular, a loss of specific microbes, including bacteria (Lactobacillus, Bifidobacteria, Bacteroides, Clostridia species, and others), yeast (Saccharomyces species, aka “Brewer’s Yeast) and helminths (worms) that co-evolved with humans and perform important immunoregulatory functions (Murdaca 2021). These microbes are called “Old Friends”. When children are raised in “hygienic” environments with low exposure to these microbes, especially in the context of the Western Diet which doesn’t support microbial diversity and the presence of Old Friends (Murdaca 2021), their immune systems do not develop in a way that supports a balance between inflammation/defense against pathogens and tolerance to food antigens or commensal microbes.
Microbes regulate gut immune cells
The key requirement for immune cells in the gut is not only to respond effectively to threats such as pathogens and toxins, but to also be “tolerant” of food antigens and beneficial microbes. Commensal microbes help maintain this balance, and the principal way they do that is by influencing phenotypes or activity (McCoy 2018) of T cells and dendritic cells/macrophages. Basically, they affect whether our immune cells behave in a pro-inflammatory or regulatory and anti-inflammatory manner and when our immune cells are active versus inhibited.
Importantly, these immune cell populations exhibit “plasticity”, meaning that they can switch back and forth between phenotypes (Sica 2012, Wang 2020), depending on the conditions in the gut. Influencing this plasticity is an important way that microbes control gut inflammation and tolerance to foods. Although all of the mechanisms they use have not been identified, it seems that ultimately whether the gut is tolerating food antigens or is intolerant and inflamed depends on the balance of activity of certain immune cells called Treg and TH17 cells. Treg cells support tolerance to food antigens, whereas TH17 cells induce inflammation. The phenotypes and activities of these cells’ activity are controlled in large part by the signals the T cells receive from microbes (Omenetti 2015). This implies that one way that the pro-inflammatory effects of stress and/or a poor diet on the gut can be ameliorated by changing to diets supporting beneficial “Old Friends”.
How do “Old Friends” help maintain tolerance and constrain inflammation?
“Old Friend” bacteria tend to be GABA and butyrate producers. Immune cells, including Tregs, TH-17, and macrophages express GABA receptors, and the effects of GABA are anti-inflammatory (Prud’hoome 2015). Butyrate, produced by “Old Friends” such as bifidobacteria and certain Clostridia species, seems to be an important signal to induce Treg cells, and inhibit pro-inflammatory TH17 cells (Chen 2018). Tryptophan metabolites generated by microbes can also induce Tregs (Langgartner 2019). The ways that “Old Friend” helminths (worms, such as Heligmosomoides polygyrus) can induce tolerance are less clear but seem to involve programming macrophages, dendritic cells, T cells, and B cells to a regulatory phenotype (Rook 2014). Pre-clinical studies investigating the effects of probiotic bacteria (e.g. lactobacillus species and the VSL3 combination of eight “Old Friends”) support the idea that these “Old Friends” do indeed program gut immune cells (Rook 2014).
“Old Friends” help the gut barrier
Gut barrier permeability, or “leaky gut” is a consistent pathological feature associated with dysbiosis, which implies that some of the “missing microbes” are normally helping to maintain gut barrier integrity. This is likely a principal mechanism by which microbial populations containing “Old Friends” can support resilience to stress, and thus reinforces the importance of a diet that supports these “Old Friends”.
Because many “Old Friend” bacteria, including Bifidobacteria, Clostridium clusters IV and XIVa, and others, produce butyrate, they play a pivotal function in gut barrier health. This is because butyrate serves as the principal source of energy for the gut epithelial cells. Indeed, these bacteria provide approximately 80% of the energy needs for the epithelial cells. Thus, butyrate-producing “Old Friend” bacteria may prevent or help heal damage to the gut barrier by ensuring these cells have adequate energy to perform their functions.
In addition, butyrate can influence the expression of the junction proteins that bind the epithelial cells and act to reduce permeability. Support for the idea that butyrate-producing bacteria can help heal the gut comes from studies of fecal transplant donors. Some donors, called “Super Donors” provide material that is more effective for curing C. difficile infections than material from other donors (Wilson 2019). Characterizing the material from the Super Donors has found that this material contains more of the “Old Friend” Clostridium clusters IV and XIVa, which are known to be good butyrate producers (Wilson 2019).
The mucin layer is a key component of the gut barrier, and degraded mucin is associated with increased permeability and inflammation (Engevik 2019, Sicard 2017). Notably, mucin degradation is a key pathophysiological feature of inflammatory bowel disease. Microbes play a role in maintaining its integrity, by several mechanisms including butyrate production and influence on mucin production by the goblet cells (Engevik 2019). Mucin is made up mostly of protein protected by a carbohydrate, glycan. Microbes live on the mucin layer and some form biofilms. It is clear that these microbes have a symbiotic relationship with the mucin. The microbes can digest and metabolize mucin carbohydrates and glycoprotein, basically feeding on the mucus, and in turn, act to protect the mucus/gut barrier (Paone 2020, Sicard 2017).
Does keeping a healthy microbe population lead to a longer life?
Although there is still much to learn about the specific ways that microbes contribute to both health and disease, some themes are emerging from studies of people living in regions where in which many people live long, healthy lives. These regions have been dubbed “Blue Zones”, and studies have examined the microbial populations in long-lived centenarians, or people who live beyond age 100, from these Blue Zones (Sato 2021, Tuikhar 2019). These studies provide important clues regarding what it takes to establish and maintain a healthy gut and microbial population. The patterns of microbe populations in centenarians are similar across different geographical areas (Tuikhar 2019). One of the themes is that Blue Zone centenarians have microbe populations that are enriched in butyrate-producing bacteria (Tuikhar 2019) that provide energy for the gut lining cells, as well as bacteria that are believed to help protect the mucin layer of the gut barrier. Similarly, Blue Zone centenarians have higher levels of the anti-inflammatory neurotransmitter GABA and the presence of GABA-producing bacteria (Tuikhar 2019, suggesting that microbial-produced GABA may be helping to maintain the gut barrier and the immune system in a tolerant, un-inflamed state.
Although there are thousands of different microbial species that can inhabit humans, most healthy people living in “Westernized” countries have fewer different species than those living in non-industrialized regions. Many people, due to antibiotic use, food additives, pesticides, food, and generally poor nutrition, have low microbial diversity (Murdaca 2021). The urbanized Western lifestyle includes much more “hygienic” living conditions than the rural lifestyles typical of developing non-“Westernized” countries, and thus there are fewer opportunities for interaction with microbes.
This, along with vaccinations, has dramatically reduced the load of infectious diseases but is associated with an apparently consequent and rather dramatic increase in allergies, autoimmune diseases, and inflammatory bowel disease (IBD) (Murdaca 2021). In support of this idea, “Blue Zone” communities are typically rural and people eat a traditional, diverse diet. Studies of centenarians from several different countries, including India, China, Japan, and Italy, have demonstrated that unlike elderly people in Westernized cultures, who usually have less diversity in their microbial populations compared to younger people, these healthy, very elderly people have greater diversity (Tuikhara 2019).
Microbes and maternity
Not only do gut microbes play pivotal roles in gut health, but they also contribute to healthy pregnancies (Socha-Banasiak 2021). Microbes support pregnancy by providing important nutrients that support metabolic needs and may play a role in immune tolerance as well (Socha-Banasiak). This is important because the immune system plays a key role in many aspects of pregnancy, including implantation of the fetus in the womb, tissue changes in the cervix, and the timing of onset of labor (Yockey 2018). The immune system must stay in a tolerant state, in order to avoid rejecting the fetus. But the immune system must also be able to prevent infections that could damage the baby or induce pre-term labor. It is a delicate balance. The likely benefits of a healthy microbe population underline the importance of a good diet during pregnancy.
We used to think that babies are sterile until they are born, but that is not the case, as a few microbes colonize babies in the womb (Gagliardi 2018). The type of delivery, Caesarian section or vaginal, determines the immediate population of bacteria that colonize babies (Mei 2019). This difference may not be important in the long term, however, because the populations of microbes change immediately when babies begin to eat and are modified continuously until they are 1-3 years old (Yatsunenko 2012). Breast milk contains beneficial microbes, notably Bifidobacteria (Socha-Banasiak 2021), so whether or not babies are nursed may be a more important factor than delivery type in determining early infancy microbe populations.
Pre-natal microbes may play a role in neurodevelopmental disorders such as autism. Both gut microbe and immune abnormalities are seen in autistic individuals and their mothers (Li 2019, Mei 2019), which is significant because the immune system plays important roles in support of developing brains. Interestingly, studies in animals have implicated microbes in a variety of developmental functions, including “wiring” of the fetal brain, emotionality, and cognition (Dinan 2015). The precise link between microbes and brain development is not established, but some evidence suggests microbial influence on immune system function. One idea is that the disordered microbes in the mother may program her immune system in such a way that it compromises certain features of brain development in her child (Dinan 2015).
Bidirectional relationship between microbes and stress
Thanks to the close connections of the gut and the brain, the effects of psychological stress are very often felt in the gut. Microbes modulate stress responses in the gut by influencing the balance of tolerance versus immunity, by their influence on the gut barrier, and by their interactions with interoceptive nerves that influence mood states, activity, emotion, and cognition (Langgartner 2019). The actions of some microbes, typically pathobionts, can contribute to or exacerbate stress, whereas other microbes seem to confer resilience (Langgartner 2019). The resilience-promoting microbes are typically the “Old Friends” who rely on a varied diet of fiber-containing foods. One of the deleterious effects of stress is biased food choices towards fatty, sugary, low-fiber processed foods that do not favor the bacteria that can mitigate stress responses.
Stress is associated with the reorganization of microbial populations and the loss of “Old Friends” such as Lactobacillus species (Langgartner 2019, Rook 2014). Stress-related hormones can directly influence pathobiont bacteria via the hormone receptors they possess (Lyte 1992, Moriera 2016), leading to increased growth in numbers of the bacteria, disruption of the balance with other species (dysbiosis), and increased virulence (the ability of a microbe to cause disease). Increased virulence means increased release of toxins and increased “adherence”, sticking to the mucous or epithelial cell layer. This “adherence” can induce or enhance inflammation and disturb gut barrier function. Together this induces inflammation, and disruption in the balance in Treg/TH17 cells, which can reduce tolerance to food antigens. In this way, “top-down” effects of stress can predispose to food allergies, insensitivities, and other bowel disorders that are associated with gut barrier dysfunction and inflammation. In addition, pro-inflammatory cytokines generated during gut inflammation can activate the interoceptive vagus nerve pathway, contributing to mood disorders, pain, and the negative emotions associated with stress.
One dramatic example of stress and dysbiosis is necrotizing enterocolitis, (NEC), a life-threatening inflammatory condition of the gut which can develop in premature infants. This condition can be helped by treatment with bifidobacteria (Aceti 2015). In a pre-clinical model of NEC, bifidobacteria down-regulated a substance called zonulin, which is associated with increased gut permeability (leaky gut), and reduced expression of pro-inflammatory cytokines (Ling 2016). Bifidobacteria are normally found in breast milk. These findings reinforce the importance of microbes in gut barrier health and emphasize the importance of breastfeeding of infants (when possible – a fed baby is best).
Fermented foods: Ancient pro-biotics
Fermented foods, such as yogurt, kefir, cheese, sauerkraut, kombucha, beer, etc., have been a part of the human diet for thousands of years (Gasparrini 2016). It has been estimated that sheep, cattle, and goats were domesticated more than 10,000 years ago, and there are records of fermented dairy products from ancient Sumerian texts dating to 2500 BC. In the chapter Genesis of the Hebrew Torah and Christian Bible (18:8), when the Lord visits Abraham, he is offered “curds”, a type of fermented milk or cheese, and these ancient books include later references to “curds” as well. Evidence of fermented beverages has been found in China, dating to 7000 BC, and in Mesopotamia dating to 5000 BC. There are references to the health benefits of fermented dairy for gastrointestinal infections in Ayurvedic texts, and a Persian translation of Genesis is said to report that Abraham used “curds” for gastrointestinal problems. Later, Pliny the Elder, a Roman in the second century AD, recommended thick, fermented, sour milk for gastrointestinal infections (Gasbarrini 2016). Interestingly, the naturally occurring microbes in fermented foods such as Greek yogurt and kefir typically belong to the “Old Friends” group.
The idea that microbes are responsible for the health benefits of fermented foods was first noted by Elie Metchnikoff, a Nobel Laureate for his work in immunology, in the late 1800s. He had become concerned with lifestyle and aging and attributed the longevity of Bulgarian country people to their prized yogurt, from which he had isolated Lactobacillus species including one he named L. bulgaricus (Gasparrini 2016). As these people often lived to be centenarians, they were perhaps the first “Blue Zone” population identified. Metchnikoff came to believe that disease and aging were caused by bacterial toxins released into the colon, and that benefits of fermented foods are that they replace toxic bacteria with beneficial ones.
The term “probiotic” was coined in the 1960s, but it was not until high-throughput gene sequencing technology was applied to the human microbiome to identify microbial genes, that the association of microbial diversity and dysbiosis with health and disease became clear. Metchnikoff’s idea that certain “beneficial” microbes can prevent or mitigate the effects of dysbiosis on GI disorders and other conditions is now being tested by both basic science and clinical studies.
Is there an evidence base for using probiotics to help gut conditions?
As yet, there is no clear consensus on the benefits of probiotics for specific conditions. This is partly because the interpretation of studies is difficult due to differences in probiotics tested and other methodological inconsistencies. In practice, there is no regulation or guaranteed quality control for commercially available probiotic formulations. This makes it hard to apply findings from carefully controlled studies using verified formulations to the real-world availability of such formulations.
That said, probiotic preparations that contain strains of “Old Friends”, such as Lactobacillus, Bifidobacteria and Saccharomyces, as well as high fiber diets that support Old Friends (next section), have been reported fairly consistently to confer benefits for GI problems such as “traveler’s diarrhea”, irritable bowel syndrome, and mood in both animals and people (Dale 2019, Mc Farland 2019, Park 2018, Simon 2021, Wastyk 2021).
Because inflammatory bowel diseases are associated with dysbiosis and gut barrier dysfunction, probiotics might be expected to be helpful. However, findings from studies of probiotics for Crohn’s Disease and ulcerative colitis have been mixed. Probiotics do seem to be helpful for ulcerative colitis, but not so much for Crohn’s Disease. The reasons for the difference may be related to genetic factors affecting responses to different bacteria or foods (Caio 2021).
Microbes depend on our diet: All food is “pre-biotic”
Our specific populations of microbes are unique to us as individuals. Although genetic factors may influence which microbes inhabit our gastrointestinal system, environmental factors seem to be the most important determinants of microbial populations in our guts. These factors include whether we have pets, with whom we end up sharing microbes, the people we live with, our history of antibiotic use, but most especially, our diets (Davis 2016). The dependence of microbe populations on our diet is demonstrated by studies in laboratory animals and people showing that changes in diet can have dramatic effects on the composition of microbial populations. For example, changing from a meat-based diet to a plant-based diet complexly reorganizes our microbe population (David 2014) as does bariatric surgery, which also requires a change in diet. What we eat provides microbes with the kinds of foods that they can metabolize (ferment). Thus, our overall diet has “pre-biotic” functions. Broadly speaking, “prebiotics” are any kind of nutrient that microbes can metabolize, thus they support the “care and feeding” of our gut microbe
That diet plays such an outsize role in microbial diversity was a bit of a surprise, most microbes live in the colon, and thus they ferment foods, such as fibers, that we cannot digest or absorb. Until recently, the only way to study gut microbes was to culture them in test tubes from feces, and not that many different kinds of microbes are able to grow this way. The ones that could be studied fermented a restricted set of non-digestible fibers, such as inulin, a commercially available fiber “pre-biotic” supplement. But it is clear now that the types of food substances that can support microbes are far more diverse. In addition, we now know that there are microbe populations throughout the gut, including the mouth, esophagus, stomach, and small intestine (Martinez-Guryn 2019). They are harder to study than microbes from the colon, which can be isolated non-invasively from feces., These microbes are exposed to food before it is digested and thus likely are fermenting pretty much whatever it is we eat.
One implication of the prebiotic nature of most foods involves “biotransformation” of dietary antioxidants. The structure of many dietary antioxidants makes them difficult for the gut to absorb. This low bioavailability has led to skepticism regarding the true benefits of dietary antioxidants. However, some gut microbes are able to metabolize these molecules into smaller, more easily absorbed versions (Maria Monagas 2010). In this way, a diverse microbial population interacts with a diet rich in antioxidant fruits and vegetables to support health and mitigate inflammation.
Gut Advice for healthy microbes:
Eat a diverse diet- Because diet is such an important factor regulating microbial growth, diversity of food is also critical for our own diets. For instance, the Western Diet has been linked repeatedly to a wide variety of poor health conditions, all of which are associated with dysregulated inflammation (Christ 2019). The Western Diet consists of mostly only a few types of food, such as refined carbohydrates like sugar and starchy foods. This refined carbohydrate diet can lead to overgrowth of certain yeast and bacteria that can survive on that diet, and discourage growth of the many other species that prefer fiber and other components of a fresh plant-based diet. Overgrowth of yeast and bacteria causes dysbiosis, bloating, and symptoms of Irritable Bowel Syndrome (IBS) and Small Intestinal Bacterial Overgrowth (SIBO) such as cramping and pain (Saffouri 2019). This dysbiosis can contribute to inflammation at the gut barrier and leaky gut. Leaky gut can induce or exacerbate problems elsewhere in the body. The lack of “Old Friends” that normally regulate inflammation in the gut can further contribute to GI pathology as well as systemic conditions.
Avoid drastic “exclusion diets”- Exclusion diets are associated with reduced microbial diversity (Caio 2021). This can lead to dysbiosis and inflammation, and worsen symptoms.
Plant- based, “Flexitarian” or Mediterranean diets patterns are the most effective for supporting microbes and gut health (Caio 2021). Multiple lines of evidence, including epidemiological, cross-sectional and clinical trials indicate that diets that include plenty of fiber from whole grains, fruits, and vegetables support a diverse microbiome, lower incidence of mood disorders and less inflammation (Wastyk 2021).
Be mindful of chemicals in foods. Antibiotic and pesticide residues in food can metabolized by microbes (Koppel 2017). This can affect their growth, potentially contributing to dysbiosis, and some may be toxic to the microbes as well. This is one of the most compelling reasons to eat organic foods.
Avoid antibiotics, including “sanitizers”, unless they are truly necessary. Antibiotic overuse is an important contributor to the development of dysbiosis, with resistant C. difficile infections being an extreme example of what high doses of antibiotics can do. Avoid anti-biotic soaps. However, if your doctor prescribes you antibiotics, take the entire regimen!
Manage stress. Stress hormones can act as growth factors for some gut bacteria, potentially contributing to dysbiosis. Stress also leads to emotional eating (Klatzkin 2019, Zellner 2006), which is more likely to involve Western Diet-type foods that encourage low diversity and/or dysbiosis. In addition, chronic stress can contribute to increased gut barrier permeability (Hattay 2017). This “leaky gut” can drive inflammation and immune responses to foods and thus food allergies or sensitivities. These cause reduction in the diversity of diet, and consequently reduction in the diversity of microbes, contributing to dysbiosis.
Key ideas
- Our microbial populations provide important benefits for us, including making vitamins, providing fuel for gut epithelial cells, and helping us digest plants.
- A healthy population of gut microbes is one that is diverse, in that there are many different species. Having lots of different species seems to prevent any one of them from overgrowing.
- Low microbial diversity is associated with dysbiosis, which is when one or a few species of microbes overgrows. Dysbiosis can lead to symptoms such as gassiness and bloating, irritable bowel syndrome, and gut barrier inflammation, and is associated with many chronic diseases including diabetes and other metabolic diseases, cardiovascular disease, autoimmune diseases, and neurodegenerative and neurodevelopment disorders
- Healthy microbial populations are also important in pregnancy and infancy.
- There is a notable connection between gut microbe populations, dysbiosis, and brain health.
- The main influence on the types of microbial species we harbor and how much they grow is what we eat. Thus, diet is key to a healthy microbial community.
- The most important way that we can keep a diverse, balanced microbe population is by eating a varied diet of whole foods, especially whole grains and vegetables, high fiber foods such as beans and legumes, and probiotic foods. Highly processed foods that may contain toxins such as pesticides and additives that can disrupt our microbes should be avoided, as should other low fiber/low nutrition Western diet foods.
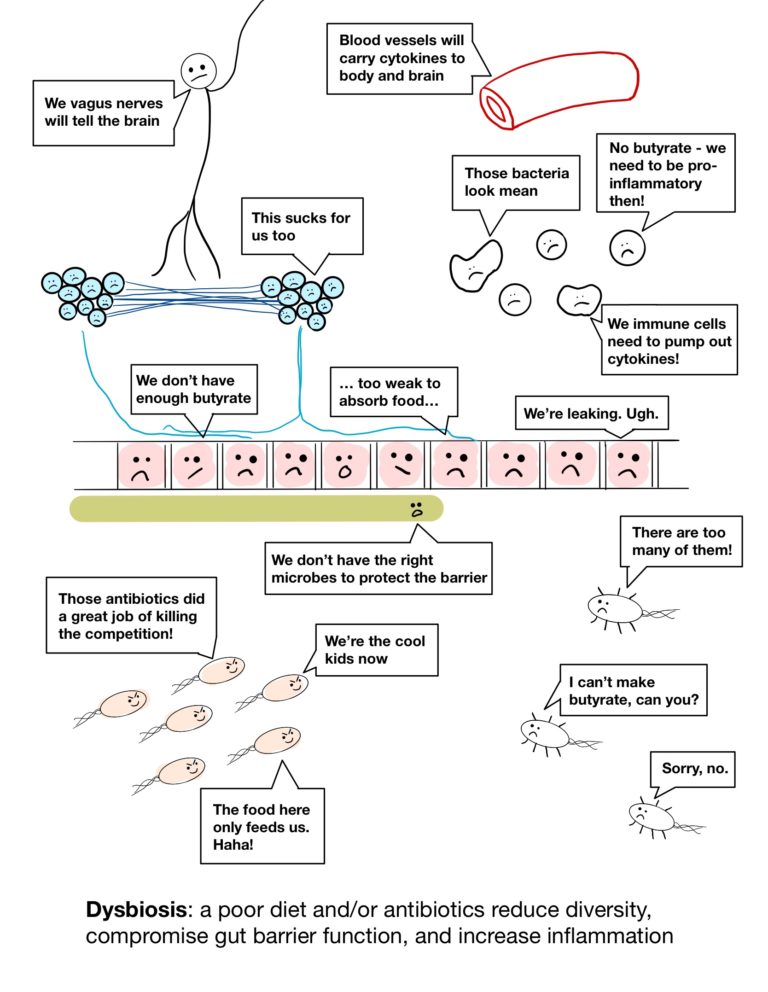
References
Aceti A, Gori D, Barone G, Callegari ML, Di Mauro A, Fantini MP, et al. Probiotics for prevention of necrotizing enterocolitis in preterm infants: systematic review and meta-analysis. Italian Journal of Pediatrics, 41:89, 2015
Bastiaanssen TFS, Cowan CSM, Claesson MJ, Dinan TG, Cryan JF. Making sense of… the microbiome in psychiatry. International Journal of Neuropsychopharmacology, 22:37-52, 2019
Bramorska A, Zarzycka W, Podilecka W, Kuc K, Brzezicka A. Age-related cognitive decline may be moderated by frequency of specific food products consumption. Nutrients, 13:2504, 2021
Caio G, Lungaro L, Capito F, Zoli E, Giancola F, Chiarioni G, De Giorgio R, Zoli G. Nutritional treatment in Crohn’s Disease. Nutrients, 13,, 1628, 2021
Camilleri M. Leaky gut: mechanisms, measurement and clinical implications in humans. Gut, 68:1516-1526, 2019
Chen J, Vitetta L. Inflammation-modulating effect of butyrate in the prevention of colon cancer by dietary fiber. Clinical Colorectal Cancer, 17:e54-544, 2018
Christ A, Lauterbach M, Latz E. Western diet and the immune system: an inflammatory connection. Immunity Review, 51:794-811, 2019
Dale HF, Rasmussen SH, Asiller OO, Lied GA. Probiotics in irritable bowel syndrome: an up-to-date systematic review. Nutrients, 11:2048, 2019
David LA, Maurice CF, Carmody RN, Gootenberg DB, Button JE, Wolfe BE, et al. Diet rapidly and reproducible alters the human gut microbiome. Nature, 505:559-563, 2014
Davis S, Yadev JS, Barrow AD, Ronertson BK. Gut microbiome diversity influences more by the Westernized dietary regime than the body mass index as assessed using effect size statistic. Microbiology Open, 6:e476, 2016
de Morales ACF, Fernandes GR, da Silva IT, Almeida-Pititto B, Gomes EP, da Costa Pereira A, Ferreira SRG. Enterotype may drive the dietary-associated cardiometabolic risk factors. Frontiers in Cellular and Infection Microbiology, 7:47, 2017
Dickerson F, Severance E, Yolken R. The microbiome, immunity, and schizophrenia and bipolar disorder. Brain, Behavior, and Immunity, 62:46-54, 2017
Dinan TG, Stilling RM, Stanton C, Cryan JF. Collective unconscious: How gut microbes shape behavior. Journal of Psychiatric Research, 63:1-9, 2015
Engevik MA, Luk B, Chang-Graham AL,Hall A, Herrmann B, Ruan W, Endres BT, Shi Z,Garey KW, Hyser JM, Versalovic J. Bifidobacterium dentium fortifies the intestinal mucus layer via autophagy and calcium signaling pathways. mBio 10:e01087, 2019
Firth J, Veronese N, Cotter J, Shivappa N, Hebert JR, Ee C, Smith L, Stubbs B, Jackson SE. Sarris J. What is the role of dietary inflammation in severe mental illness? A review of observational and experimental findings. Frontiers in Psychiatry, 10:350, 2019
Fitzgerald E, Murphy S, Martinson HA. Alpha-synuclein and the role of the microbiota in Parkinson’s Disease. Frontiers in Neuroscience. 13:369, 2019
Gasbarrini G, Bonvicini F, Gramenzi A. Probiotics History. Journal of Clinical Gastroenterology, 50, Supp. 2: S116-S119, 2016
Gagliardi A, Totino V, Cacciotti F, Iebba V, Neron Bi, Bonfiglio G, et al. Rebuilding the gut microbiota ecosystem. International Journal of Environmental Research and Public Health, 15:1679, 2018
Goehler LE, Lyte M, Gaykema RP. Infection-induced viscerosensory signals from the gut enhance anxiety: implications for psychoneuroimmunology. Brain, Behavior, and Immunity, 21:721-726, 2007
Gonzalez-Aranciba C, Urrutia-Pinones J, Illanes-Gonzales J, Sotomayor-Zarate R, Julio-Pieper M. Bravo JA. Do your gut microbes affect your brain dopamine. Psychopharmacology, in press 2019
Hattay P, Prusator DK, Tran L, Greenwood-Van Meerveld B. Psychological stress-induced colonic barrier dysfunction: Role of immune-mediated mechanisms. Neurogastroenterology and Motility, 29:e13043, 2017
Jiang TT, Shao T-Y, Ang G, Kinder JM, Turner LH, Pham G, Whitt J, Alenghat T, Way SS. Commensal fungi recapitulate the protective effects of intestinal bacteria. Cell Host & Microbe, 22:809-816, 2017
Kelly JR, Kennedy PJ, Cryan JF, Dinan TG, Clarke G, Hyland NP. Breaking down the barriers: the gut microbiome, intestinal permeability and stress-related psychiatric disorders. Frontiers in Cellular Neuroscience, 9:392. doi: 10.3389/fncel.2015.00392, 2015
Klatzkin RR, Dasani R, Warren M, Cattaneo C, Nadel T, Nikodem C, Kissileff HR. Negative affect is associated with increased stress-eating for women with high perceived stress. Physiology and Behavior, 210:112630, 2019.
Kong, F, Deng F, Ying Lic Y, Zhao J. Identification of gut microbiome signatures associated with longevity provides a promising modulation target for healthy aging. Gut Microbes, 10: 210–215, 2019
Koopman M, El Aidy S. Depressed gut? The microbiota-diet-inflammation trialogue in depression. Current Opinion in Psychiatry, 30:369-377, 2017
Koppel N, Maini Rekdal V, Balskus EP. Chemical transformation of xenobitics by the human gut microbiota. Science, 356:eaag 2770, 2017
Kumamoto CA, Greenigt, Hube B. The gut, the bad and the harmless: Candida albicans as a commensal and opportunistic pathogen in the intestine. Current Opinion in Microbiology, 56:7-15, 2021
Langgartner D, Lowry CA, Reber SO. Old Friends, immunoregulation, and stress resilience. Pflügers Archive – European Journal of Physiology, 471:237–269, 2019
Li N, Yang J, Zhang J, Liang C, Wang Y, Chen B, et al. Correlation of gut microbiome between ASD children and mothers and potential biomarkers for risk assessment. Genomic Proteomics Bioinformatics, 17:26-38, 2019
Li Y, Lu M-R, Wei Y-J, Sun L, Zhang J-X, Zhang H-G, Li B. Dietary patterns and depression risk: A meta-analysis. Psychiatry Research, 253:373-382, 2017
Ling X, Linglong P, Weixia D, Hong W. Protective Effects of Bifidobacterium on Intestinal Barrier Function in LPS-Induced Enterocyte Barrier Injury of Caco-2 Monolayers and in a Rat NEC Model. PLoS ONE 11: e0161635, 2016
Lyte M, Ernst S. Catecholamine-induced growth of Gram-negative bacteria. Life Sciences, 50:203-212, 1992
Lyte M, Varcoe JJ, Bailey MT. Anxiogenic effect of sun-clinical bacterial infection in mice in the absence of overt immune activation. Physiology and Behavior, 65:63-68, 1998
Lyte M. Microbial endocrinology and the microbiota-gut-brain axis. Advances in Experimental Medicine and Biology. 817:3-24, 2014
Lyte M. Microbial endocrinology: and ongoing personal journey. In M Lyte (ed) Microbial Endocrinology: Interkingdom Signaling in Infectious Disease and Health, Advances in Experimental Medicine and Biology 874: 1-24, 2016
Maes M. Evidence for an immune response in major depression: A review and hypothesis. Progress in Neuro-Psychopharmacology 19:11-38, 1995
Margolis KG, Cryan JF, Mayer EA. The microbiota-gut-brain axis: from motility to mood. Gastroenterology 160:1486-1501, 2021
Maria Monagas M, Urpi-Sarda M, Sanchez-Patan F, Llorach R, Garrido I, Gomez-Cordoves C ,Andres-Lacueva C, Bartolome B. Insights into the metabolism and microbial biotransformation of dietary flavan-3-ols and the bioactivity of their metabolites. Food & Function, 1: 233–253, 2010
Martinez-Guryn K, Leone V, Chang EB. Regional dicersity of the gastrointestinal microbiome. Cell Host Microbe, 26:314-324, 2019
McCoy KD, Ignacio A, Geuking MB. Microbiota and Type 2 immune responses. Current Opinion in Immunology, 54:20-27, 2018
McFarland LV, Goh S. Are probiotics and prebiotics effective in the prevention of travellers’ diarrhea: a systematic review and meta-analysis. Travel Medicine and Infectious Disease. 27:11-19, 2019
Mei C, Yang W, Wei X, Wu K, Huang D. The unique microbiome and innate immunity during pregnancy. Frontiers in Immunology, 10:2886, 2019
Moreira CG, Russell R, Mishra AA, Narayanan S, Ritchie JM, Waldor MK, Curtis MM, Winter SE, Weinshenker D, Sperandio V. Bacterial adrenergic sensors regulate virulence of enteric pathogenis in the gut. mBio 7: pii: e00826-16. 2016
Morris MC, Wang Y, Barnes LL, Bennett DA, Dawson-Hughes B, Booth SL. Nutrients and bioactives in green leafy vegetables and cognitive decline. Neurology, 90:e214-e222, 2017
Murdaca G, Greco M, Borro M, Gangemi S. Hygiene hypothesis and autoimmune disease: A narrative review of clinical evidences and mechanisms. Autoimmunity Reviews, 20:102845, 2021
Musumeci S, Coen M, Leidi A, Schrenzel J. The human gut mycobiome and the specific role of Candida albicans: where do we stand, as clinicians? Clinical Microniology and Infection, 28:58-63, 2022
Nguyen TT, Kosciolek T, Eyler LT, Knoght R, Jeste DV. Overview and systematic review of studies of microbiome in schizophrenia and bipolar disorder. Journal of Psychiatric Research, 99:50-61, 2018
Omenetti S, Pizarro TT. The Treg/Th17 Axis: A Dynamic Balance Regulated by the Gut Microbiome. Frontiers in Immunology, 6:639, 2015
Paone P, Cani PD. Mucus barrier, mucins, and the gut microbiota: the expected slimy partners? Gut, 69:2232-2243, 2020
Park C, Brietzke E, Rosenblat JD, Musial N, Zuckerman H, Ragguett R-M, et al. Probiotics for the treatment of depressive symptoms: An anti-inflammatory mechanism? Brain, Behavior, and Immunity. 73:115-124, 2019
Patterson MJ, Oh S, Underfill DM. Host-microbe interactions: commensal fungi in the gut. Current Opinion in Microbiolgy,40:131-137, 2017
Prud’homme, GJ, Glinka Y, Wang Q. Immunological GABAergic interactions and therapeutic applications in autoimmune diseases. Autoimmunity Reviews 14:1048–10, 2015
Rea K, Dinan TG, Cryan JF. The microbiome: A key regulator of stress and neuroinflammation. Neurobiology of Stress, 4:23-33, 2016
Rees T, Bosch T, Douglas AE. How the microbiome challenges our concept of self. PLoS Biology, 16:e2005358, 2018
Rinninella E, Raoul P, Franceschi F, Miggiano GAD, Gasbarrini A, Mele MC. What is the healthy gut microbiota composition? A changing ecosystem across age, environment, diet, and disease. Microorganisms, 7:14, 2019
Rogers AWL, Tsolis RM, Bäumler AJ.. Salmonella versus the microbiome.
Microbiology and Molecular Biology Reveiws, 85:e000, 2021
Rook GAW, Charles L. Raison, and Christopher A. Lowry. Microbiota, Immunoregulatory Old Friends and Psychiatric Disorders. In M. Lyte and J.F. Cryan (eds.), Microbial Endocrinology: The Microbiota-Gut-Brain Axis in Health and Disease. Advances in Experimental Medicine and Biology 817, Springer New York, 2014
Roshchina VV. New trends and perspectives in the evolution of neurotransmitters in microbial, plant and animal cells. In M Lyte (ed) Microbial Endocrinology:Interkingdom Signaling in Infectious Disease and Health, Advances in Experimental Medicine and Biology 874: 25-77, 2016
Rothschild D, Weissbrod O, Barkan E, Kurilshikov A, Korem T, Zeevi D, Costea PI, et al.. Environment dominates over host genetics in shaping human gut microbiota. Nature, 555:210-228, 2018
Saffouri GB, Shields-Cutler RR, Chen J, Yang Y Lekatz HR, Hales VL, et al. Small intestinal microbial dysbiosis underlies symptoms associated with functional gastrointestinal disorders. Nature Communications, 10:2012, 2019
Sarkodie EK, Zhou S, Baidoo SA, Chu W. Influences of stress hormones on microbial infections. Microbial Pathogenesis 131:270-276, 2019.
Sato Y, Atarashi K, Plichta DR, Arai Y, Sasajima S, Kearrney SM, ey al. Novel bile acid biosynthetic pathways are enriched in the microbiome of centenarians. Nature, 599:458-464, 2021
Sica A, Montovani A. Macrophage plasticity and polarization: in vivo veritas. The Journal of Clinical Investigation, 122:787-795, 2012
Sicard J-F, Le Bihan G, Vogeleer P, Jacques M, Harel J. Interactions of Intestinal Bacteria with Components of the Intestinal Mucus. Frontiers in Cellular and Infection. Microbiology. 7:387.doi: 10.3389/fcimb.2017.00387, 2017
Simon E, C ̆alinoiu LF, Mitrea L, Vodnar DC. Probiotics, Prebiotics, and Synbiotics: Implications and Beneficial Effects against Irritable Bowel Syndrome. Nutrients, 13:2112, 2021
Socha-Banasiak A, Pawlowska M, Czkwianianc E, Pierzynoiwska K. From intrauterine to extraunterine- the role of endogenous and exogenous factors in the regulation of the intestinal microbiota community and gut maturation in early life. Frontiers in Nutrition, 8:696966, 2021
Stilling RM, van de Wouw M, Clarke G, Stanton C, Dinan TG, Cryan JF. The neuropharmacology of butyrate: The bread and butter of the microbiota-gut-brain axis? Neurochemistry International, 99:110-132, 2016
Strandwitz P, Kim KH, Terekhova D, Liu JK, Sharma A, Levering J, McDonald D, Dietrich D, Ramadhar TR, Lekbua A, Mroue N, Liston C, Stewart EJ, Dubin MJ, Zengler K, Knight R, Gilbert JA, Clardy J, Lewis K. GABA Modulating Bacteria of the Human Gut Microbiota. Nature Microbiology, 4: 396–403. doi:10.1038/s41564-018-0307-3, 2019
Subramoni S, Venturi V. LuxR-family “solos”: bachelor sensors/regulators of signaling molecules. Microbiology, 155:1377-1385, 2009
Tian J, Dang H, Wallner M, Olsen R, Kaufman DL. Homotaurine, a safe blood-brain barrier permeable GABAA-R-specific agonist, ameliorates disease in mouse models of multiple sclerosis. Scientific Reports, 8:16555 | DOI:10.1038/s41598-018-32733- 2018
Tuikhara N, Keisam S, Labala RK, Imrata L, Ramakrishnand P, Cha Arunkumar M, Ahmed G, Biagi E, Jeyaram K. Comparative analysis of the gut microbiota in centenarians and young adults shows a common signature across genotypically non-related populations. Mechanisms of Aging and Development, 179:23-35, 2019
Vitetta L, Vitetta G and Hall S. Immunological Tolerance and Function: Associations Between Intestinal Bacteria, Probiotics, Prebiotics, and Phages. Frontiers in Immunology, 9:2240.doi: 10.3389/fimmu.2018.0, 2018
vom Steeg LG, Lein SL. Sex steroids mediate bidirectional interactions between hosts and microbes. Hormones and Behavior, 88:45-51, 2017
Wang J, Chen W-D, Wang Y-D. The relationship between gut microbiota and inflammatory diseases: The role of macrophages. Frontiers in Microbiology, 11:1065, 2020
Wastyk HC, Fragiadakis GK, Perelmean D, Dahan D,Merrile BD, Yu FB, et al. Gut-microbiota-targeted diets modulate human immune status. Cell, 184:4137-4153, 2021
Weiss GA, Hennet T. Mechanisms and consequences of intestinal dysbiosis. Cellular and Molecular Life Sciences, 74:2959-2977, 2017
Wilson BC, Vatanen T, Cutfield WS, O’Sullivan JM. The super-donor phenomenon in fecal microbiota transplantation. Frontiers in Cellular and Infection Microbiology, 9:2, doi: 10.3389/fcimb.2019.00002, 2019
Wu L, Zeng T, Zinellu A, Rubino S, Kelvin DJ, Carru C. A cross-sectional study of compositional and functional profiles of gut microbiota in Sardinia centenarians. mSystems, 4:e00325-19, 2019
Xu Z, Knight R. Dietary effects on human gut microbiome diversity. British Journal of Nutrition, 113:S-1-S5, 2015
Yang B, Wei J, Ju P, Chen J. Effects of regulating intestinal microbiota on anxiety symptoms: A systematic review. General Psychiatry.32:e100056, 2019
Yatsunenko T, Rey FE, Manary MJ, Trehan I, Dominguez-Bello MG, Contreras M, et al. Human gut microbiome viewed across age and geography. Nature, 486:222-227, 2012
Yocker LJ Iwasaki A. Role of interferons and cytokines in pregnancy and fetal development. Immunity, 49:397-412, 2018
Zellner DA, Loaiza S, Gonzalez Z, Pita J, Morales J, Pecora B, Wolf A. Food selection changes under stress. Physiology and Behavior, 87:789-793, 2006.